Designer genes
Geneticist Nicola Dennis scans the world of genetic modification, mostly outside New Zealand.
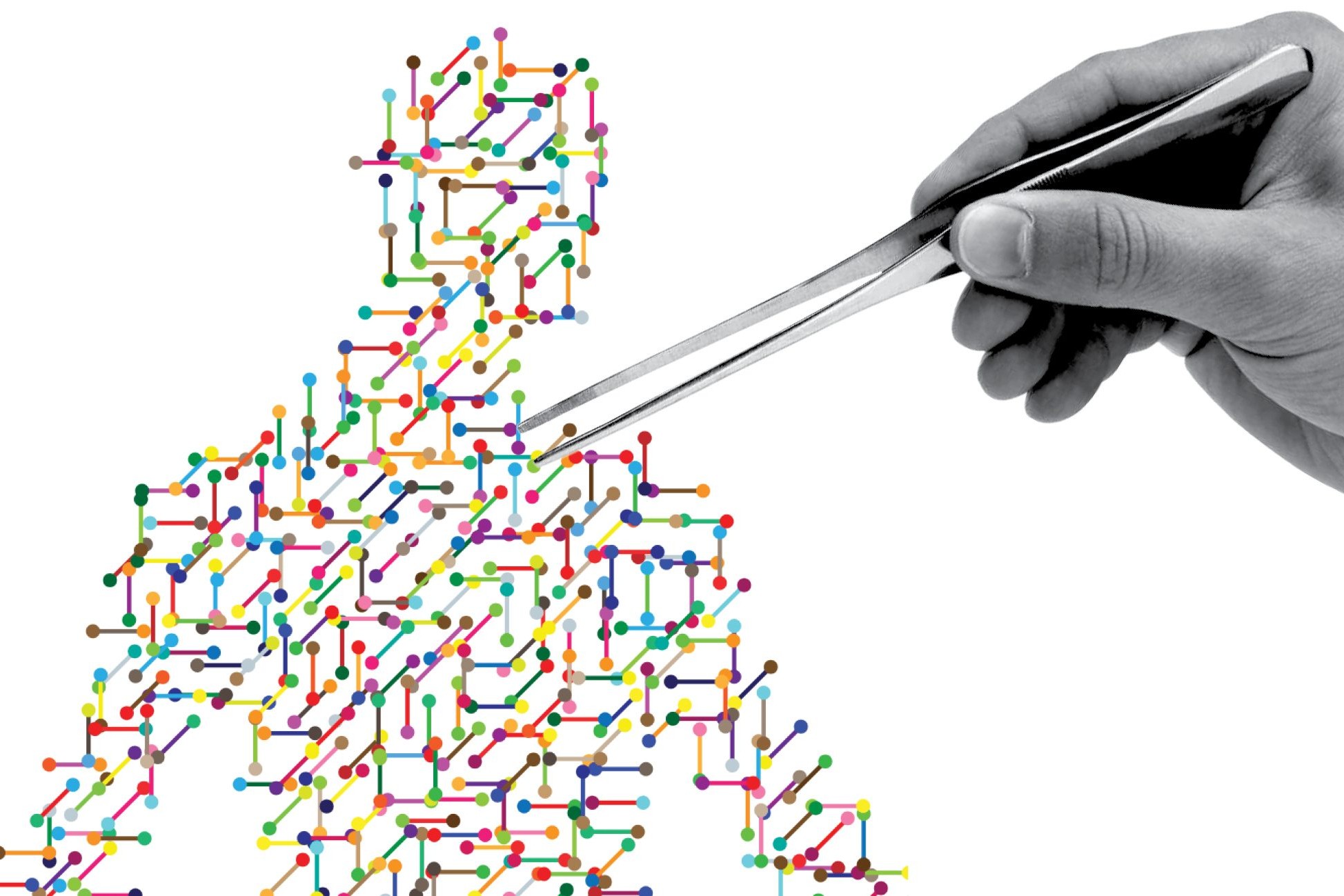
Geneticist Nicola Dennis scans the world of genetic modification, mostly outside New Zealand.
Genetic modification, genetic engineering, gene editing. It’s fair to say the science of tinkering with genes in the genome has had a fair bit of rebranding over the years.
The marketing has yet to resonate with the New Zealand authorities who won’t even entertain the thought of letting it out of the lab and into general circulation. It seems a little unfair that all the other countries get it, but we miss out.
But what is it, really? Other than something the man won’t let us have.
What is genetic modification?
To the purists, even your bog-standard breeding programme is genetic modification (GM). Generations of selective breeding has turned the wolf into a Dachshund.
There is an argument to be made for classifying yappy little dogs as genetically modified organisms.
But, of course we are usually referring to the speedier kinds of modification. Scientific meddling with DNA. Popping genes in, taking them out, inserting/fixing mutations.
Genetic engineering/genetic modification are often used as the umbrella terms for this practice, although you will occasionally find people who will make very boring distinctions between the two.
Whereas, gene editing is a newer, more targeted approach for making small changes to an existing gene (i.e. to fix a disease causing mutation). You might also hear the term CRISPR which is kind of like the Sellotape/sticky tape situation of the genetic modification world. CRISPR is a technique that has become so popular that its name is often used interchangeably with gene editing.
To understand the fanfare of CRISPR we have to look back on some horrible history.
Some horrible history
Back in the olden days (the 1990s), there was some pretty crude genetic modification going on. It involved bombarding cells with DNA that encoded a gene and some extra bits to help drive it.
This franken DNA was usually delivered with a modified virus if the target was a cell that was already part of someone. But, if we are talking about a lonesome cell yet to turn into a plant or animal (when making a germline mutation), then it might involve electricity or firing particles at it. There wasn’t a tonne of precision about where in the cell’s genome the gene ended up which ended up causing some heartbreak.
The highest priorities at the time were:
- Testing that genes could actually be transplanted from one species to another (i.e. transgenics) and
- Trying to fix children who had inherited very serious genetic mutations (i.e. gene therapy).
The latter involved children with severe combined immunodeficiency (SCID or “the boy in the bubble” disease), where children born with a faulty cytokine gene lack a working immune system.
In total, 20 children were given a virus containing the missing cytokine gene. Seventeen of the children were cured of their SCID. But not without tragedy.
Shotgunning a genome with DNA is a recipe for causing cancer, since the insertion of the new gene can upset the delicate regulation of other genes if it lands somewhere inappropriate.
Five of the children developed leukaemia.
Gene therapy looked promising, but it was far too risky without the technology to control where the genome was affected (that precision would later be delivered by CRISPR).
While gene therapy quietly buried its mistakes, transgenic plants carried the flag boldly. It’s not so daunting if you accidentally give plants tumours. Just pick the plant (or a piece of it) that didn’t develop any issues and you have your new transgenic breeding line.
Golden rice, Flavr Savr tomatoes, blue roses, and a bunch of other commercially dismal genetically modified plants were put before the very reluctant public.
Most wacky plants were harshly rejected, but the glyphosate-resistant trait was well received. In glyphosate-resistant crops, weeds could be controlled with the inexpensive and relatively harmless glyphosate rather than dipping into the heavier chemistry often required for selective control.
You are forbidden from growing GM crops here in NZ, but chances are you have consumed them. Imported foods made from herbicide-resistant canola, corn, rice, soybeans and sugar beet are all authorised for sale in NZ, provided some perfunctory food labelling is in place.
CRISPR – for controlling where you mutate
Whether or not genetic modification is abhorrent to you kind of depends on your level of trust in your fellow humans. But we can probably all agree that if you are going to do it, then focusing your meddling on only your targeted location should be a bare minimum requirement. Scientists experimented with enzymes designed to cut DNA at certain places (i.e. site-specific nucleases) to try to take some creative control over their mutations. But a much simpler system was found in an unlikely place. It came from a proper “what the hell is this” analysis of weird repeating DNA sequences found in bacterial genomes. There were clusters of these repeating sequences interspaced with bits of DNA that looked like it came from other organisms. It was the DNA equivalent of a crop circle.
Scientists called this phenomenon Clustered Regularly Interspaced Short Palindromic Repeats or CRISPR for short. We now suspect that this is the bacterial equivalent of an immune system.
The bacteria senses an invading virus is trying to hijack its genetic material, it cuts up the virus’s genetic code, takes a piece of the virus’s genetic code and makes itself a new gene dedicated to cutting up that virus next time it sees it again.
In 2012, scientists worked out how to modify this system for introducing precise genetic modifications. Or gene editing as it was now called, to put some distance between this new system and the one that killed children and produced peculiar plants.
Although this kind of breezes over the fact that CRISPR is not limited to “editing” existing genes. CRISPR is also used for popping in genes from other organisms to make transgenic creatures.
Gene editing livestock and plants
There was a public whoopsy when United States company, Recombinetics, tried to introduce the polled (hornless) mutation into non-polled cattle. The idea would be that top genetic dairy sires could be nipped and tucked into losing their horns so that no genetic progress for production would be lost in the pursuit of a polled dairy herd.
A fine idea that should definitely be developed further. However, the FDA discovered that the genome of Buri, the experimental bull produced, was contaminated with bacterial DNA.
Buri and his progeny were packing a bacterial gene for antibiotic resistance. The kind of gene that would have been used to help grow the machinery needed for the gene editing. An error that could have been easily avoided had the company properly verified that they weren’t exposing their cattle embryos to anything but the desired genetic sequence.
On a more positive note, Japan seems to have successfully commercialised CRISPR-modified tomatoes and fish. The tomatoes have five times the normal level of a gamma-aminobutyric acid, a compound that has a vague health halo polished by marketing. The fish are two smallish species (the Red Sea Bream and the Tiger Puffer) modified to grow 1.2-1.9 times larger than normal.
Genetic modification in NZ
Closer to home, AgResearch has developed a genetically modified rye grass with a higher fat content. This boosts the energy content of the pasture and could reduce the amount of drymatter grazing livestock have to eat to get their fill.
It could be a useful tool for reducing agricultural methane emissions. It might also never be allowed in the NZ outdoors. Research grazing trials had to be conducted in the US as they were not permitted here. Those trials should be complete by now, but things have gone very quiet.
Meanwhile the science scene is aflutter with scientists researching “gene drives” for pest control. The idea is that you modify a lab-bound pest with an unfavourable mutation that would slow their population growth (e.g. knocking out the gene used to make male offspring). Along with this unfavourable gene you also include the genes for the CRISPR technology.
When a mating takes place out in the wild (assuming you are allowed to release the GM pest), the CRISPR machinery modifies any natural copies of the gene that it encounters to include the new mutation. This means that any matings between a mutant and a wildtype pest result in 100% mutant offspring. Theoretically, anyway.
I can imagine a scenario where the last male standing has a CRISPR-resistant mutation that rocks back through the population with vengeance. Or maybe something not all that useful to humans mutates inside the pest’s gene drive system and then we have a mutant population of poison-resistant rats that can pick locks.
Existing challenges (where the scientists play)
Outside of my wild imagination, there are some notable limitations for the modern gene editing system. Not being allowed to have an adult conversation about its use in NZ is a major one. But, like all emergent technologies the hype is blustering over some important limitations.
First you can’t, yet, mutate any piece of DNA you choose. The CRISPR machinery is looking for specific patterns on the DNA to prove it is where it thinks it is – remember it thinks it is in a bacteria fighting off viruses. So there has to be some tomfoolery going on with the protein machinery to trick it into performing the desired behaviour.
The genomic site of the mutation you want to introduce or change might not be suitable for this kind of trickery. Or it might not be unique enough. With CRISPR you have a small amount of sequence (20ish base pairs) with which to identify your target address and CRISPR is not too fussed if it doesn’t line up exactly.
If you have a genomic address that reads like “10 Beach Street” you might end up bombing some unintended targets. And of course, a lot of paper is devoted to working out the best way to safely deliver the CRISPR machinery into living cells. Preferably with some precision and dignity if we are talking about gene therapy in ailing humans.
Viruses are a bit old school and can lead to unintended (read deadly) inflammatory responses. However, there have been some very recent breakthroughs in using nanoparticles to deliver genetic material (e.g. the covid mRNA vaccine) to cells. So we can expect the hamsters to be pounding the wheel steadily in that direction.
Gene editing people
CRISPR and a few of its lesser-known cousins have put gene therapy back on the medical research scene. There have been some very promising cancer trials where CRISPR has been used to modify the immune system to fight off advanced cancer.
There are trials for curing a common form of inherited childhood blindness, for editing transplant cells to cure type 1 diabetes, and just last month a NZ man had his liver genetically modified to lower his cholesterol.
I am being a bit click-baity here, the man had a genetic disorder where cholesterol levels get so high that you can literally see it in the sufferer’s eyes.
At the moment, delivering the CRISPR mishmash of bacterial protein and synthetic RNA into the human body is proving a challenge. So for gene therapy, this new technology is usually focused on easy to get to targets like the blood and the liver.
That isn’t so much of a problem for germline mutations where you are treating a single cell or an embryo. Scientists are legally allowed to mess with human embryos for research purposes, but there is a global understanding that you must not grow them out.
Designer babies are very bad for the brand. An overeager Chinese scientist earned himself a (confusingly short) three-year prison sentence for creating a pair of CRISPR babies. He was aiming to make the twins HIV-resistant in preparation for their life with a HIV-positive parent. It didn’t work, the desired mutation was not widespread throughout the children’s cells, and the whole experiment was condemned as unethical and reckless
Glossary
Cell: The small Lego pieces of your body. Each cell holds a copy of your genetic code.
CRISPR: A new fangled way limiting genetic modifications to a particular site in the genome. Helpful for reducing collateral damage.
DNA: The chemical that your genetic code is made of.
Gene: A sequence of DNA that holds the instructions for a particular protein or sometimes other non-protein things.
Gene drive: Modifying a genome (probably via CRISPR) so that it contains the CRISPR machinery. The idea is that the inherited CRISPR machinery keeps on messing with the genome to ensure all offspring (and future descendents) inherit the unfavourable genes. Remember that movie Inception, it’s like the pest control version of that.
Gene editing: A more modern way of genetic modification which only makes small changes in existing genes.
Gene therapy: Repairing disease causing mutations via genetic modification. Still in experimental stages in human medicine. Only allowed under strict conditions in consenting children/adults, not in embryos who can’t give consent.
Genetic modification/engineering: Changing genes in a cell or person/ creature. These are general terms for adding genes, disabling genes, fixing or causing mutations.
Genome: The word that means “all the genetic information in a cell”. It is a concept rather than a specific place because genes are spread out over multiple chromosomes etc.
Germline modification: Modifying an individual cell or embryo in the hopes of turning it into a living breathing thing with the modification in every one of its cells. Allowed in plants and animals, but considered a step too far for humans.
Nanoparticles: Tiny little particles that almost have no relevance here.
Organism: A broad term of any living thing. I prefer to use the word “creature” because it is less likely to be mistaken for the bedroom climax.
Transgenics: Taking a gene (i.e. herbicide-resistance) out of one organism (i.e. Agrobacterium) and putting it into another (i.e. soybeans).